GENETICS: Using DNA Hybridization to Compare the DNA of Different Organisms; Polymorphic Genes and Types of Mutation.
DNA image by Pete Linforth from Pixabay
The technique of DNA hybridization can be used to compare the similarity of the DNA of different species, and is proving to be a useful tool in the search to piece together evolutionary trees.
DNA is much more stable than protein because it is held together by regular hydrogen bonds along the whole of its length. However, when DNA is heated to about 87 °C, known as the melting point of DNA, the hydrogen bonds break and the two halves of the molecule separate. When the temperature drops below 87°C, the two strands join up: they re-anneal. This is how the basic process of DNA hybridization works:
- DNA from two different species is cut into small fragments and heated to 87 °C so that the two strands separate. The separated strands from each species are then mixed together. As the temperature drops, the DNA strands re-anneal. Some of the molecules formed will be hybrids: they contain one strand from each species.
- The temperature at which the hybrid strands re-anneal tells us about the degree of similarity between the species. When two strands from the same species re-anneal, they do so at 87 °C. Closely related DNA re-anneals at temperatures slightly lower but close to 87 °C. This indicates that they have many base sequences in common and so form many hydrogen bonds along the length of the molecule. In contrast, DNA from more distantly related species must cool to much lower temperatures before they can anneal as there are fewer hydrogen bonds to hold the strands together.
For example, human DNA can be hybridized with gorilla DNA or rabbit DNA. The melting point for DNA from all three single species is 87 °C. The melting point for the human/rabbit DNA is lower than for the human/gorilla DNA. This shows that humans and gorillas have more DNA sequences in common than humans and rabbits.
POLYMORPHIC GENES
Genes that have two or more alleles are called polymorphic. Many genes have no alleles – they code for essential proteins and any mutation in them is selected against.
For instance, the genes that code for the enzymes in respiration must be copied exactly time after time, or the organism dies. Some genes, however, can cope with a bit of variation. The genes that code for eye colour or hair colour are obvious examples. In humans, it has been estimated that 31.7 per cent of our genes are polymorphic.
MUTATIONS
A mutation is a spontaneous change in the genetic material of an organism. It usually occurs when DNA is copied and cells divide. Generally, there are two types of mutation: a gene mutation and a chromosome mutation.
Both of these may occur in somatic cells (normal body cells) or germ cells (sex cells). Somatic cell mutations are not passed on to the next generation. Generally, organisms accumulate somatic mutations as they get older, and this is thought to be one of the causes of cancer. In contrast, when a mutation arises in an egg or sperm cell which then goes on to become a zygote, the mutation is passed on to all the cells of the new individual. Germ cell mutations alter the genome of an organism.
Gene mutations
A gene mutation is a change in the base sequence of DNA. We have already seen that when DNA replicates, two identical strands are formed. Occasionally, however, a fault can occur. In a typical gene of, say, 1400 base pairs, a change in just one of the base pairs has the potential to make the whole gene useless, and can prove lethal to the organism. A change in a single base is known as a point mutation.
How can such a tiny change be so disastrous? It is because a change to any base will alter a codon so that it will probably code for a different amino acid. In turn, this ‘wrong’ amino acid can affect the way the polypeptide chain folds, so changing the shape of the whole protein molecule. It might then be unable to function. (Remember: many genes code for enzymes or parts of enzymes whose proper functioning relics on precise shape.) The table below shows the different types of gene mutation that can occur, using words instead of codons. In all cases, the original meaning of the message is lost.
Different types of gene mutation
Type of gene mutation | Effect on code (using words rather than codons) |
normal code | THE FAT OLD CAT SAW THE DOG |
addition (frame shift) | THE EFA TOL DCA TSA WTH EDO G |
deletion (frame shift) | THE ATO LDC ATS AWT HED OG |
substitution | THE FAT OLD BAT SAW THE DOG |
duplication | THE FAT TFA TOL DCA TSA WTH EDO G |
inversion | THE TAF OLD CAT SAW THE DOG |
From the table above, we can see that the two least damaging types of mutation are substitutions and inversions, because these alter only one of the seven amino acids. In some cases, if the new amino acid occurs in a non-vital part of the chain, or has similar properties to the correct one, then the mutation might not actually matter at all.
In contrast, deletions and additions are potentially much more damaging because they cause frame shifts: only one base may be added or lost, but this causes the whole sequence to shift along, altering all the codes. In this case, the protein made is completely different from the original and is unlikely to function as it should. Later on in this post, I will discuss the sickle cell anaemia, a disease caused by a fault in just one base.
How common are gene mutations?
The rate at which genes mutate varies between species and between genes at different loci. At a rough estimate, there is an average mutation rate of about 10-5 per locus per generation, meaning 1 in 100,000. So, in a population of one million individuals, you could expect ten to have inherited a mutation at any particular gene. The rate of gene mutation (and chromosome mutation) can be greatly increased by environmental factors, known as mutagens, such as ultraviolet, X-rays, alpha and beta radiation, and chemicals such as mustard gas and cigarette smoke.
Chromosome mutations
During mitosis (normal cell division) chromosomes are copied, condensed and pulled apart. The end result should be that each daughter cell receives a complete set of perfect chromosomes. But faults can occur. A chromosome mutation occurs when the structure of a whole chromosome – or set of chromosomes – is altered in some way. The figure below shows the basic types of chromosome mutation: deletion, duplication, inversion and reciprocal translation.
While gene mutations normally affect just one gene, chromosome mutations involve the disruption of whole blocks of genes. For this reason, many chromosonal mutations give rise to syndromes, which are complex sets of symptoms with a single underlying cause.
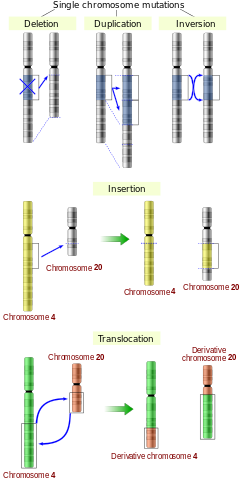
In addition to the structural mutations outlined above, another common failure is non-disjunction. This occurs when pairs of chromosomes fail to separate during anaphase I of meiosis. Some gametes get both chromosomes, while others receive neither. Down’s syndrome, for example, occurs when a gamete containing two copies of chromosome 21 joins with a normal gamete containing one, giving the affected individual three copies of chromosome 21.
Sickle cell anaemia
A small minority of people, particularly those of African origin, inherit sickle cell anaemia (SCA), a condition in which their haemoglobin acts abnormally. SCA is a genetic disease: it results from a gene mutation that is inherited. Carriers of one sickle cell allele are usually perfectly healthy, and in some circumstances even have an advantage over people who possess two normal alleles. However, individuals with two sickle cell alleles have the disease.
The faulty allele makes abnormal haemoglobin S instead of the normal haemoglobin. Problems arise when oxygen tensions are low, as they are in actively respiring tissues. At low oxygen tensions, the abnormal haemoglobin tends to come out of solution and crystallise, distorting the red blood cells into crescent shapes. This has two main consequences. First, sickle cells tend to form clots, which can block blood vessels. Secondly, the spleen destroys abnormal sickle cells at a much greater rate than normal, causing anaemia.
Sickle cell anaemia. BruceBlaus, CC BY-SA 4.0
Haemoglobin molecules consist of four polypeptide chains: two α-globins and two β-globins. DNA analysis shows that sickle cell anaemia results from a fault in just one base in the β-globin gene. The base sequence of the first seven codons of this gene reads:
Normal base sequence: GUA-CAU-UUA-ACU-CCU-GAA-GAG
Sickle cell sequence: GUA-CAU-UUA-ACU-CCU-GUA-GAG
You can see that there is a point mutation (or substitution) in the sixth codon. This tiny change leads to a different amino acid being built into the protein, one that changes its shape and properties.
The inheritance of sickle cell anaemia
Generally, people have two copies of each gene, and this is the case with sickle cell anaemia. The two alleles involved are:
HbA = coding for normal haemoglobin
HbS = coding for sickle cell haemoglobin
So the three possible genotypes are:
HbAHbA: Normal
HbAHbS: Carriers of the disease but with few, if any, symptoms
HbSHbS: Have the disease
The sickle cell gene is far commoner than you would expect for one which is selected against. The reason for this is that the carriers usually show a higher resistance to malaria than normal. So while HbAHbA individuals are susceptible to malaria, and HbSHbS individuals tend to die from sickle cell anaemia, HbAHbS individuals have neither disadvantage and are more likely to survive and reproduce. Hence the HbS allele does not disappear from the gene pool.
Polyploidy
Occasionally, a mutation causes a whole set of chromosomes to be changed. For instance, a fault in meiosis might result in some gametes having two sets of chromosomes, while others receive none. When such a diploid gamete joins with a normal one, the result is a triploid zygote – one with three sets of chromosomes. In another case, a fault in mitosis after fertilization could result in the chromosomes doubling but not separating, leaving four sets in a single tetraploid cell. Neither of these embryos could develop.
The possession of multiple sets of chromosomes is known as polyploidy. This phenomenon is very common in flowering plants and has played a vital role in their evolution. Botanists estimate that over half of the world’s flowering plant species are polyploid, including some of the world’s most economically important crops: sugar beet, tomatoes and tobacco.
Why mutations are important
A mutation can have one of several effects:
- It can be lethal: an organism with a lethal mutation cannot survive and might not even develop beyond the zygote. Genes code for vital proteins such as enzymes, so many gene mutations mean that the protein is the wrong shape, and so cannot function metabolically. Chromosome mutation which involve the loss of whole blocks of genes are usually lethal.
- It can have no effect. It might occur in a non-coding part of DNA, or in a gene that is not expressed. Alternatively, it can result in a different amino acid being incorporated into the protein, but one that does not alter its ability to function.
- It can be beneficial. Occasionally, a mutation produces an improvement in phenotype. Statistically, this is a very rare event, but given the number of DNA replications and the number of individual organisms, it is bound to happen sooner or later. Beneficial mutations are hugely important because, ultimately, they are the source of all variation. Natural selection favours these ‘improved’ mutants.
Following the Industrial revolution, a
mutation in the peppered moth produced a black (melanic) form that was better
camouflaged against sooty backgrounds. The black moth had an advantage because
it was hard for predators to see and so survived to pass on its genes to the
next generation. Consequently, the mutated gene spread and the genome of the
species was altered.
CONCLUSION
After reading my posts on genetics from GENETICS: Resveratol, GENETICS: The role of RNA in transfer and this last post, you should now know and understand that:
A chromosome is a large, densely staining body consisting of a single, supercoiled DNA strand. The genes along a chromosome are found at particular points called loci. A gene is a length of DNA that codes for the synthesis of a particular polypeptide or protein. Only about 10 per cent of a chromosome consists of genes; the rest is non-coding DNA. The genetic code is the sequence of bases contained in the DNA molecule. The code is read in groups of three bases, called codons. Each codon codes for a particular amino acid. The central dogma (principle) of molecular biology is that genes are read by messenger RNA which in turn moves out into the cytoplasm, where it codes for the assembly of a protein.
You should have also known that at the ribosome, messenger RNA attracts transfer RNA molecules with the right amino acids attached. The amino acids are held together so that peptide bonds form; in this way the protein is assembled. DNA replication occurs when the two strands separate and the enzyme DNA polymerase assembles two new strands on the originals. This is called semi-conservative replication as each old strand is conserved as half of the new DNA.
Finally, a fault in DNA replication leads to a gene mutation. The base sequence is altered so that the gene no longer codes for the correct protein. Chromosome mutations can result in whole blocks of genes being lost, or translated backwards for example. And most mutations are harmful, some have no effect, but a few can be beneficial. Beneficial mutations might give the organism an advantage; in this way, mutations can give rise to variation and hence drive evolution.
Thanks for coming.
REFERENCES
https://www.britannica.com/science/polymorphism-biology
https://en.wikipedia.org/wiki/Gene_polymorphism
https://www.sciencedirect.com/topics/biochemistry-genetics-and-molecular-biology/gene-mutation
https://en.wikipedia.org/wiki/Mutation
https://ghr.nlm.nih.gov/primer/mutationsanddisorders/genemutation
https://phys.org/news/2018-04-genetic-mutations-common-thought.html
https://ghr.nlm.nih.gov/primer/mutationsanddisorders/structuralchanges
https://en.wikipedia.org/wiki/Chromosome_abnormality
https://www.thoughtco.com/types-of-chromosome-mutations-1224525
https://www.sciencedirect.com/topics/medicine-and-dentistry/chromosome-mutation
https://medlineplus.gov/sicklecelldisease.html
https://www.mayoclinic.org/diseases-conditions/sickle-cell-anemia/symptoms-causes/syc-20355876
https://en.wikipedia.org/wiki/Sickle_cell_disease
https://www.medicinenet.com/sickle_cell/article.htm
https://sickle.bwh.harvard.edu/scd_inheritance.html
https://sicklecellanemianews.com/inheritance-sickle-cell-anemia/
https://rarediseases.info.nih.gov/diseases/8614/sickle-cell-anemia
https://www.sciencedirect.com/topics/agricultural-and-biological-sciences/polyploidy
Congratulations @loveforlove! You have completed the following achievement on the Steem blockchain and have been rewarded with new badge(s) :
You can view your badges on your Steem Board and compare to others on the Steem Ranking
If you no longer want to receive notifications, reply to this comment with the word
STOP