SUPPORT AND MOVEMENT: How the Human Body Moves.
A simple action, such as tapping your finger on the table, involves the skeletal system, the muscular system and the nervous system. This voluntary action begins as a stimulus in the cerebrum of the brain. Motor nerves carry impulses to muscles and cause them to contract, pulling on bones through the tough inelastic tendons.
Muscles can only pull, or contract: they cannot push. This means that muscles rarely act alone: most of the time they work in groups. Contraction of a muscle moves a bone at a joint, but a second (antagonistic) muscle returns the bone to its original position. Take movement in the arm, for example. The biceps muscle bends or flexes the arm; the triceps muscle straightens or extends the arm.
We all know that machines can help us to lift heavy loads using levers. The bones in our bodies also act as levers. The principle of leverage allows a muscle to overcome the resistance supplied by a weight. This happens in three ways:
- An arm bends because a force is applied between the pivot (the elbow) and the resistance (the weight to be lifted).
- We stand on tiptoes by pivoting the toes on the ground and using our calf muscles to raise the weight at the ankles. This is the wheelbarrow principle.
- When standing or sitting, we can raise our head because at the back of the neck tip the skull at the pivot at the top of the spine. This is the seesaw principle.
The ‘stuck finger’ test demonstrates the effect of muscles working in groups. Each of your fingers has a separate tendon connecting it to muscles in the forearm. Try it. Curl up your middle finger and place the other four fingers on a hard surface. Now lift up the other fingers one by one. You will find that you can lift all of your fingers except the ring finger. This is because these two fingers share the same tendon connection.
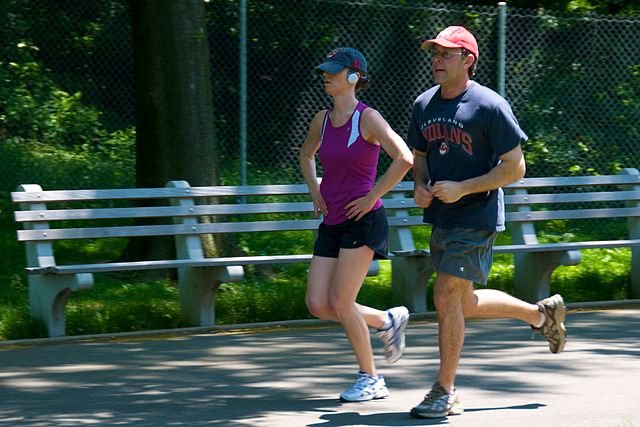
MUSCLES AND MOVEMENT
The bones of the human skeleton provide a basic system of levers and joints that makes the skeleton potentially movable, but neither levers nor joints can move without muscles. There are three types of muscle. Skeletal muscles are responsible for whole-body movement. Smooth muscle is responsible for automatic movements such as those involved in peristalsis. Cardiac muscle is found only in the heart.
Skeletal muscle (also called striped or striated muscle) provides the main source of power for human locomotion. In this section, I will look at the structure and function of skeletal muscle.
THE PROPERTIES OF SKELETAL MUSCLE
Like other sorts of muscle, skeletal muscle has three basic properties:
- Excitability: it can receive and respond to a stimulus.
- Extensibility: it can be stretched and it can contract.
- Elasticity: it can return to its original shape after it has contracted.
Skeletal muscle is also described as voluntary muscle because we can contract it when we want to. Smooth muscle and cardiac muscle are involuntary muscle: we cannot consciously control its contraction.
Skeletal muscles contract and relax, moving bones at joints in the skeleton. This allows for movements such as walking, running and waving, and fine movements such as those needed to use tools. The action of skeletal muscles also helps to maintain the position of the body when we are sitting or standing even though there is no obvious movement. The contractions of skeletal muscle also produce heat, and much of this is used to keep the body temperature at a normal level.
THE CONTRACTION OF SKELETAL MUSCLE
An individual muscle is made up from hundreds of cylindrical muscle fibres, about 50 μm in diameter and ranging in length from a few millimetres to several centimetres. The muscles fibres are not composed of individual cells – the cell membranes have broken down and so each fibre has many nucIei. Each fibre is surrounded by a modified cell membrane called the sarcolemma. Each muscle fibre is composed of many long cylindrical myofibrils, consisting of a repeating arrangement of proteins which causes the banding pattern. Each repeated pattern of proteins is called a sarcomere.
The two main proteins involved in muscular contraction are actin (thin) and myosin (thick) filaments. Muscles contract when the actin fibre are pulled over the myosin fibres. Two other proteins involved are tropomyosin and troponin. Tropomyosin winds around the actin filament, preventing it from binding to myosin. Troponin (a globular protein) moves the tropomyosin out of the way, allowing actin to bind to myosin, thus initiating muscular contraction. Within each sarcomere there are two light bands; the I zone consisting of just actin filaments and the H zone that consists of just myosin. Between them are darker areas where these proteins overlap. When muscles contract, the actin and myosin filaments are pulled over each other so that the light bands get smaller. Note that the width of the dark band corresponds to the width of the myosin molecules, so it doesn’t get any narrower – molecules don’t shrink, they just slide over each other. The steps in muscular contraction are:
An impulse arrives down a motor nerve and terminates at the neuromuscular junction (a modified synapse). The synapse secretes acetylcholine. Acetylcholine fits into receptor sites on the motor end plate. This initiates an action potential in the muscle cell membrane, which then spreads throughout the myofilaments via the sarcoplasmic reticulum (modified endoplasmic reticulum) and T tubules. The action potential causes a change in the permeability of the sarcoplasmic reticulum, resulting in an influx of calcium ions into the myofilament. The calcium ions bind to the troponin, changing its shape. Troponin displaces the tropomyosin, so that the myosin heads can bind to the actin. The myosin head pulls backwards, so that the actin is pulled over the myosin. An ATP molecule becomes fixed to the myosin head, causing it to detach from the actin. The splitting of ATP provides the energy to move the myosin head back to its original position, ‘cocking the trigger’ again. The myosin head becomes reattached to the actin once again, but further along. In this way, the actin is quickly pulled over the myosin in a rachet motion, shortening the sarcomere, the whole filament and the muscle itself.
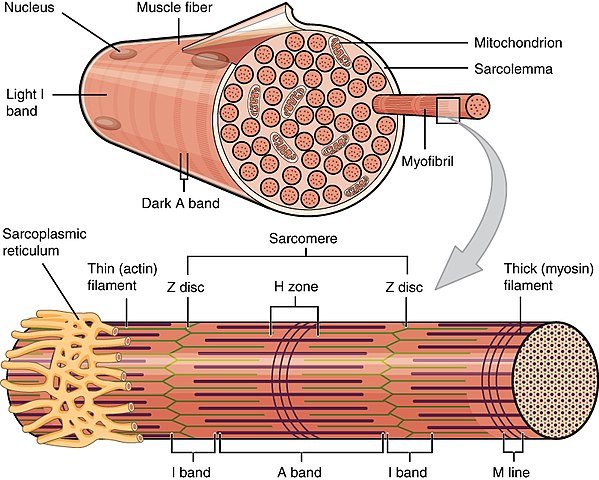
Fast and slow muscle fibres
A nerve impulse is the trigger for muscle contraction, but the length of time a contraction lasts depends on how long calcium ions remain in the sarcoplasm. This time is different in different types of skeletal muscle fibres. Fast twitch fibres and slow twitch fibres are classified on the basis of their contraction times.
There are three important differences between fast and slow fibres:
- Slow twitch fibres have less sarcoplasmic reticulum than fast twitch fibres. This means that calcium ions remain in their sarcoplasm longer.
- Slow twitch fibres have more mitochondria which provide ATP for sustained contraction.
- Slow twitch fibres have significantly more myoglobin than fast twitch fibres. Myoglobin has a higher affinity for oxygen than the haemoglobin in blood and so is particularly efficient at extracting oxygen from the blood.
A central principle in physiology is that structure is closely related to function and this is illustrated clearly by the distribution of the muscle types in the body. For instance, the postural muscles – such as those in the neck and back – have a high proportion of slow twitch fibres. In contrast, the muscles that bring about fast, explosive movements such as running, jumping and throwing are packed with fast twitch fibres.
Different people have different ratios of fast twitch to slow twitch fibres in the muscles – this is determined by the type of muscle fibres they inherit and also by the amount and type of training they have done. Marathon runners have more slow twitch muscle fibres: sprinters have more fast twitch muscle fibres.
A table showing the analysis of the average proportions muscle fibre types in selected muscles in different male athletes
Type of athlete | Approx % Fast twitch | Approx % Slow twitch |
---|---|---|
Marathon runner | 18 | 82 |
Swimmer | 25 | 75 |
Cyclist | 40 | 60 |
800 metre runner | 52 | 48 |
untrained person | 55 | 45 |
Sprinter and jumper | 62 | 38 |
Fast twitch muscle fibres can be further subdivided, those giving us three different muscle types:
Slow twitch oxidative (type I) fibres
These fibres contain large amounts of myoglobin, which is why they appear real. They also have many mitochondria and a dense capillary network. These fibre release ATP slowly by aerobic respiration. The contractions they produce may not be very powerful, but vitally there is no build-up of lactate and so the muscles can go on working for long periods.
Fast twitch oxidative (type IIA) fibres
These show similar features to slow twitch fibres: a lot of myoglobin, many mitochondria and blood capillaries: but they are able to hydrolyse ATP far more quickly and therefore to contract rapidly. They are relatively resistant to fatigue, but not as resistant as type I fibres.
Fast twitch glycolytic (type IIB) fibres
These fibres have a relatively low myoglobin content, few mitochondria and few capillaries. They contain large amounts of glycogen, which provides fuel for anaerobic ATP production via the process of glycolysis. They contract rapidly but fatigue quickly, owing to the build-up of lactic acid. Type IIB fibres appear whiter than the other two types.
TIME OF DEATH
When a body is discovered, a pathologist investigates and tries to estimate how long the person has been dead. This information can be important in a murder enquiry. The pathologist assesses the time of death, partly by taking the internal temperature, to see how much the body has cooled from the normal body temperature of 37 ˚C, and partly by looking at the state of the muscles.
When death occurs, ATP is no longer made. It is a short-lived chemical and so it runs out fairly quickly. This causes the muscles to lock into position as cross-bridges that formed between actin and myosin filaments before death can no longer be broken. This condition, rigor mortis happens in all body muscles. It appears about four hours after death and lasts about 24 hours. After this time, muscle proteins are destroyed by enzymes within the cells and so rigor mortis disappears.
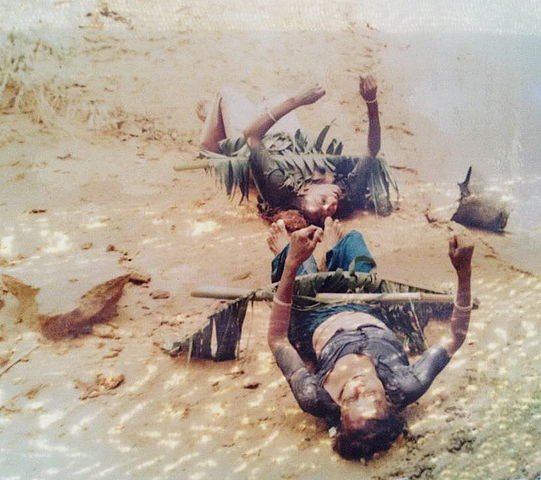
SUMMARY
After reading the previous post and then this, you should know and understand the following that:
- There are three basic types of skeleton: hydrostatic skeletons, endoskeletons and exoskeletons. Invertebrates such as earthworms have hydrostatic skeletons, arthropods and crustaceans have exoskeletons, and vertebrates, including humans, have endoskeletons. Skeletons support and protect the body and allow animals to locomote (move from place to place).
- The human skeleton is made of bones, tendons and ligaments. The human skeleton is movable because different bones meet at joints, across which muscles are attached. Because muscles can pull but not push, muscles often work in pairs called antagonistic pairs. Muscles are excitable, extensible and elastic. Skeletal muscle, also called striped, striated or voluntary muscle, is responsible for human locomotion, maintaining body posture and generating body heat. Skeletal muscle is made of muscle fibres which contain bunches of myofibrils which in turn contain myofilaments. Thick myosin filaments lie alongside thin actin filaments.
- The sliding filament hypothesis explains muscle contraction at the molecular level. The events of muscular contraction can be summarized as follows. A nerve impulse arrives at the neuromuscular junction. This releases acetylcholine, which changes the ionic permeability of the sarcolemma. Calcium ions are released into the myofilaments, where they bind to the tropomyosin-troponin-actin complex, and activate ATPase. Cross-bridges form between actin and myosin, the fibres slide over each other and the muscle contracts.
Thank you for reading.
REFERENCES
- https://wonderopolis.org/wonder/how-do-we-move
- https://kidshealth.org/en/teens/bones-muscles-joints.html#:~:text=Muscles%20move%20body%20parts%20by,a%20limb%20at%20a%20joint.
- https://training.seer.cancer.gov/anatomy/muscular/
- https://www2.estrellamountain.edu/faculty/farabee/biobk/BioBookMUSSKEL.html
- https://amactraining.co.uk/resources/handy-information/free-learning-material/level-3-exercise-and-fitness-knowledge-index/level-3-39-properties-of-skeletal-muscle/
- https://byjus.com/biology/skeletal-muscle/#:~:text=The%20skeletal%20muscles%20have%20the,to%20respond%20to%20a%20stimulus.
- https://www.ptdirect.com/training-design/anatomy-and-physiology/skeletal-muscle-the-physiology-of-contraction
- https://en.wikipedia.org/wiki/Muscle_contraction#:~:text=Muscle%20contraction%20is%20the%20activation,dumbbell%20at%20the%20same%20position.
- https://www.bbc.co.uk/science/humanbody/body/factfiles/fastandslowtwitch/soleus.shtml
- https://www.verywellfit.com/fast-and-slow-twitch-muscle-fibers-3120094
- https://blog.nasm.org/fitness/fast-twitch-vs-slow-twitch#:~:text=Fast%20Twitch%20Muscle%20Fiber%20Types,such%20as%20sprinting%20or%20weightlifting.
- https://www.encyclopedia.com/science/encyclopedias-almanacs-transcripts-and-maps/time-death
Congratulations @loveforlove! You have completed the following achievement on the Hive blockchain and have been rewarded with new badge(s) :
You can view your badges on your board and compare yourself to others in the Ranking
If you no longer want to receive notifications, reply to this comment with the word
STOP
Do not miss the last post from @hivebuzz:
Thanks for your contribution to the STEMsocial community. Feel free to join us on discord to get to know the rest of us!
Please consider supporting our funding proposal, approving our witness (@stem.witness) or delegating to the @stemsocial account (for some ROI).
Thanks for using the STEMsocial app, which gives you stronger support. Including @stemsocial as a beneficiary could yield even more support next time.