ENZYMES AND METABOLISM: The Secret of the Runny Filling In a Creme Egg.
Hello, dear readers. Did you know it is easy to see how confectioners make chocolates with hard centres: they simply pour molten chocolate over the centre and wait for it to set. But what about the soft centres? It isn’t possible to pour liquid chocolate over a liquid centre and still keep the shape. So, how do they make the runny, yolk-like mixture inside chocolate eggs?
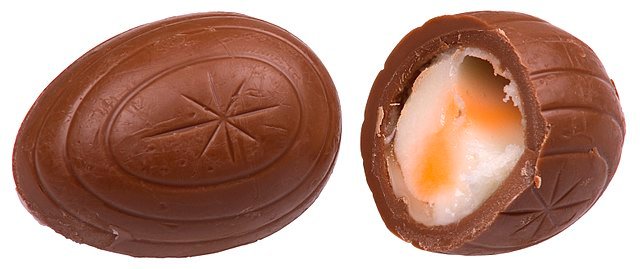
Chocolate lovers everywhere may be surprised to learn that the answer is use an enzyme. To start with, the centre is solid and contains an enzyme and a polysaccharide. After the chocolate coating has set, the enzyme breaks down the long polysaccharide chains, turning the hard centre into the familiar runny filling. Increasingly, enzymes are being used in medicine, industry and biotechnology.
WHAT ARE ENZYMES?
Enzymes are complex chemicals that control reactions in living cells. They are biochemical catalysts., speeding up reactions that would otherwise happen too slowly to be of any use to the organism. This definition is a bit of an oversimplification. An active enzyme may speed up a particular reaction, but living organisms do not need all reactions to be going at the maximum rate all of the time. The key word is control. It is more accurate to say that enzymes interact with other molecules to produce an ordered, stable reaction system in which the products of any reaction are made when they are needed, in the amount needed.
THE ROLE OF ENZYMES IN AN ORGANISM
The figure below is part of a metabolic pathway chart showing some of the large number of different but interconnected chemical reactions in a living cell. Charts like this are too complex to learn but they illustrate several important points:
- Many of the complex chemicals that living organisms need cannot be made in a single reaction. Instead, a series of simpler reactions occurs, one after another, forming a metabolic pathway. A single pathway may have many steps in which each chemical is converted to the next. A specific enzyme controls each reaction.
- Each individual step in the chart represents one of the simple chemical reactions. At first glance, there seem to be a great variety of reaction, but look more closely and you will see that the same types of reaction occur again and again.
- Enzymes control cell metabolism by regulating how and when reactions occur. Using this very simple pathway as an example,
A → B → C → D
the final product is substance D, the chemical needed by the living organism. The pathway needs three different enzymes and, when D is no longer needed, or if too much has been produced. One of the three enzymes is ‘switched off’.
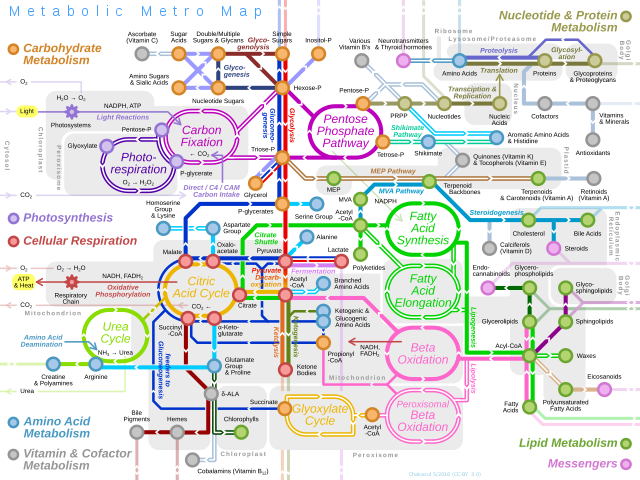
One enzyme, one reaction – but what a difference
When carbon dioxide dissolves in water, a small proportion of the carbon dioxide molecules combine with water to form carbonic acid.
CO2 + H2O →H2CO3 → H+ + HCO3-
It is a rather slow reaction, but, in the presence of the enzyme carbonic anhydrase, it is speeded up about 107 (ten million) times. One molecule of carbonic anhydrase can convert 600 000 molecules of carbon dioxide into carbonic acid every second. In living cells, this reaction allows other processes to occur much faster:
- In red blood cells, the enzyme speeds up the production of acid. Which in turn causes oxyhaemoglobin to give up its oxygen. Without the enzyme, delivery of oxygen to the tissues would be much slower.
- In certain cells in the stomach lining, the activity of carbonic anhydrase in a pathway allows hydrochloric acid to be secreted rapidly. This creates the acidic conditions necessary for digestive enzymes in the stomach to work properly.
- In the cells of the kidney tubule, carbonic anhydrase speeds up excretion of excess acid, and so helps to maintain the pH of the body at the correct level.
THE CHEMICAL NATURE OF ENZYMES
Enzymes are globular proteins. They have a very precise overall shape (tertiary structure) formed from one or more polypeptides. Usually, they are roughly spherical, hence the term globular protein. This overall three-dimensional shape of an enzyme molecule is very important: if it is altered, the enzyme cannot bind to its substrate and so cannot function. Enzyme shape is maintained by hydrogen bonds and ionic forces and enzyme function can be affected by changes in temperature and pH.
Enzymes have several important properties:
- Enzymes are specific: each one catalyses only one reaction.
- Enzymes combine with their substrates to form temporary enzyme-substrate complexes.
- Enzymes are not altered or used up by the reactions they catalyse, so can be used again and again.
- Enzymes work very rapidly and each has its own turnover number.
- Enzymes are sensitive to temperature and pH.
- Enzyme function can be slowed down or stopped by inhibitors.
THE SPECIFICITY OF ENZYMES
Taking digestive enzymes, you might find it difficult to believe that each enzyme catalyses only one reaction. Trypsin, for example, can begin the digestion of a wide variety of foods rich in protein: eggs, pork, chicken, and soya, for example. But when you look at how trypsin works at the molecular level, you can see that this enzyme is specific. Trypsin cuts an amino acid chain at a point between two particular amino acids, arginine and lysine and nowhere else. Most proteins have these two amino acids next to each other at some points in their polypeptide chain, and so can be partly digested by trypsin.
Several scientists in biochemistry have devised mechanisms often called models – to explain how enzymes work. In coming up with ideas, they have had to take account of enzyme specificity. Two models that are used to explain how enzymes work are the lock-and-key hypothesis and the induced-fit hypothesis.
The lock-and-key hypothesis
This idea assumes that enzyme function depends on an area on the molecule known as the active site. The active site is a groove or pocket in the surface of the enzyme into which the substrate molecule fits. Typically. The active site is formed by 3 to 12 amino acids. The size, shape and chemical nature of the active site correspond closely with that of the substrate molecule, so they fit together like a key fits into a lock or, perhaps more realistically, like two pieces in a three-dimensional jigsaw.
Although this model helps us to understand some of the properties of enzymes, it is now generally accepted that a modified version, known as the induced-fit hypothesis, better represents what happens when an enzyme catalyses a reaction.
The induced-fit hypothesis
Experimental evidence suggests that the active site in many enzymes is not exactly the same shape as the substrate, but moulds itself around the substrate as the enzyme-substrate complex is formed. Only when the substrate binds to the enzyme is the active site the correct shape to catalyse the reaction. As the products of the reaction form, they fit the active site less well and fall away from it. Without the substrate, the enzyme reverts to its ‘relaxed’ state, until the next substrate molecule comes along.
Both models show why enzymes are not altered by the reactions that they catalyse: they bind to a substrate momentarily, allowing a reaction to happen, but do not themselves undergo any chemical change.
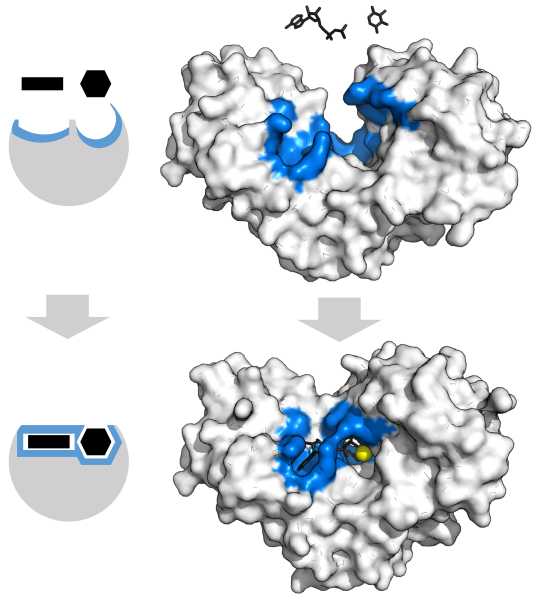
THE CHEMISTRY OF ENZYME ACTION
In biology, almost all reactions are reversible. If they were not, there would be no recycling of molecules because once large molecules had been built up they could not be broken down again.
A simple reversible reaction can be expressed as:
A + B (reactants) ⇌ C + D (products)
In this reaction, the reactants (A and B) combine to give the products (C and D). If this reaction were to take place in a test tube, a proportion of the products would react to form A and B again. Eventually, an equilibrium would be reached in which the relative proportion of reactants and products would remain the same.
In theory, an enzyme allows a reversible reaction to reach equilibrium more quickly. An enzyme can speed the reaction in either direction; the way it does so depends on whether there are more reactants or products present at the start. In living organisms, however, enzymes usually speed up reactions in one particular direction. This is because, in any organism, an enzyme acts only if the product of the reaction that it catalyses is needed. And, as the product is used as soon as it is made, equilibrium is rarely reached.
ENZYMES WORK BY LOWERING ACTIVATION ENERGY
For any chemical reaction to take place, bonds must be broken before new ones can form. The energy needed to break these bonds, and so set the reaction in motion, is the activation energy.
Many reactants need a large amount of energy to push them to a state where they can take part in a reaction, so many reactions take place only at high temperature. Many substances burn, for instance, but only after the initial activation energy has been supplied, perhaps by lighting a match.
In the presence of enzymes, the activation energy is greatly lowered and this allows reactions to take place at the relatively low temperatures normally found in living organisms. The 'halfway' point in a reaction is called the transition state, and this is represented by the top of the curve. The transition state represents the stage when the old bonds have been broken in order to allow new ones to form. Enzymes lower the activation energy by making it easier to achieve the transition state.
Enzymes speed up reactions in a number of ways:
- They can hold the substrates close together at the correct angle – this would otherwise have to occur by chance collision.
- They can position any charged groups on the active site to help the reaction to occur.
- By acid or base catalysis: the active site of the enzyme can behave as an acid or a base, donating or accepting protons (hydrogen ions, H+) from the substrate.
- Through structural flexibility: the flexible shape of the enzyme can change during catalysis. This ensures that the substrates are brought together in the correct sequence for the reaction.
Many metabolic reactions require energy in addition to the presence of the relevant enzyme. This energy is supplied by ATP made by cell respiration. Reactions that need energy are made to happen by coupling them with ATP breakdown, and so are called coupled reactions.
How fast do enzymes work?
The speed at which an enzyme works is expressed as its turnover number. This is usually defined as the number of substrate molecules turned into product in one minute by one molecule of enzyme. Values range from less than 100 to many millions. Some examples are listed in the table below:
NAMING AND CLASSIFYING ENZYMES
Older enzyme names such as pepsin, catalase and trypsin give no clues about the nature of the reaction they catalyse. To cope with the rapidly expanding number of new enzymes, the International Union of Biochemistry has developed a scheme for naming and classifying enzymes. Very generally, enzymes are named be adding the suffix -ase to the name of their substrate. The rest of the name attempts to indicate the nature of the reaction taking place. Alcohol dehydrogenase. for example, catalyses the removal of hydrogen from alcohol (ethanol). Further examples are given in the table below.
A table showing some common enzymes and their substrates
Enzyme | Substrate | Reaction catalysed |
---|---|---|
maltase | maltose | hydrolysis of maltose to glucose |
amylase | starch | hydrolysis of starch to maltose |
alcohol dehydrogenase | alcohol (ethanol) | removal of hydrogen from alcohol |
DNA ligase | DNA | joining together two DNA strands |
RNA polymerase | nucleotides that make RNA | synthesis of mRNA on a DNA molecule |
glycogen synthetase | glucose | polymerisation of glucose into glycogen |
ATPase | ATP | synthesis or splitting of ATP |
Thank you for coming.
REFERENCES
https://www.thespruceeats.com/creme-eggs-520777
https://www.livescience.com/45145-how-do-enzymes-work.html
https://www.easybiologyclass.com/enzyme-substrate-specificity-types-classification/
https://socratic.org/questions/58f64d5c11ef6b44e4d659b6
http://chemistry.elmhurst.edu/vchembook/571lockkey.html
https://www.britannica.com/science/induced-fit-theory
https://study.com/academy/lesson/induced-fit-enzyme-model-definition-theory-quiz.html
https://en.wikipedia.org/wiki/Enzyme
http://book.bionumbers.org/how-many-reactions-do-enzymes-carry-out-each-second/
https://www.ncbi.nlm.nih.gov/books/NBK22380/
https://www.quora.com/How-fast-do-enzymes-work-in-humans
https://iopscience.iop.org/book/978-0-7503-1302-5/chapter/bk978-0-7503-1302-5ch1
https://www2.estrellamountain.edu/faculty/farabee/biobk/BioBookEnzym.html
https://www.medicalnewstoday.com/articles/319704
That's a lot of work¡ Really appreciate it.
Thanks for your contribution to the STEMsocial community. Feel free to join us on discord to get to know the rest of us!
Please consider supporting our funding proposal, approving our witness (@stem.witness) or delegating to the @stemsocial account (for some ROI).
Thanks for using the STEMsocial app and including @stemsocial as a beneficiary, which give you stronger support.