Understanding the chemistry behind electric cars, using redox reactions, cells, and electrode potentials.
Hello hivers, my esteemed readers, erudite colleagues and awesome students of chemistry. It has been a while I last posted here, I am happy to be back on my feet. On my blog today, I shall be discussing the chemistry behind electric cars in relationship with redox reactions and cell potentials.
We all know that electric cars and vans would dramatically reduce atmospheric pollution. So, why haven’t they yet supplanted petrol and diesel-driven vehicles? Their widespread adoption has been stunted by the absence of a battery able to provide sufficient motive power for a sufficient length of time. Much is being done to rectify this, with the development of lightweight batteries that can run a vehicle for at least 500 km between charges. It is hoped that these batteries can be recharged many times using cheap-rate electricity.
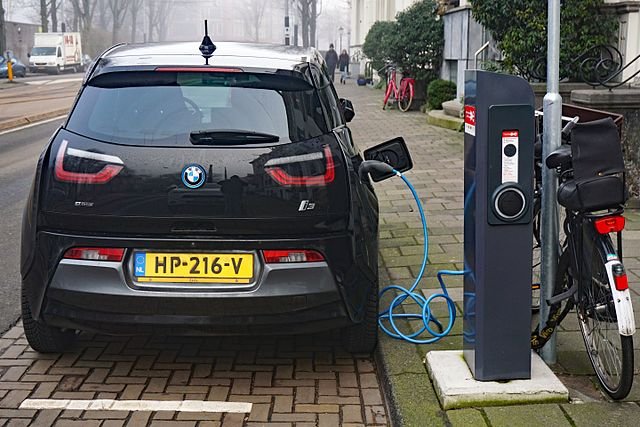
There has also been progress with the development of fuel cells that use hydrogen or petrol in a reaction with oxygen. No burning occurs and electric current is generated directly. Appropriate technology does exist, but there are still problems with the availability, storage and transport of hydrogen. All these development wouldn’t have come to place without the understanding of the chemistry behind redox reaction and cell potentials.
So, what are redox reactions?
Redox is a type of chemical reaction that involves electron transfer. At its simplest, one atom loses electrons and another atom gains electrons. Atoms of metals lose electrons, usually to attain a stable electron configuration, and atoms of non-metals gain electrons, usually to attain a stable electron configuration. In redox reactions, we make use of half equations.
A typical redox reaction is the reaction of the metal magnesium with dilute hydrochloric acid to form magnesium chloride:
Mg(s) + 2HCI(aq) → MgCl2(aq) + H2(g)
This reaction is a redox reaction because electron transfer occurs. An atom of magnesium loses two electrons during the reaction, but it is not immediately obvious where these electrons go. Hydrochloric acid is a strong acid and it is completely dissociated into aqueous chloride ions and aqueous hydrogen ions. It is the hydrogen ions that gain electrons: two hydrogen ions gain one electron each, eventually to form a hydrogen molecule. The hydrogen ions are said to be reduced to hydrogen. The chloride ions do not take part in the reaction at all. They are sometimes called spectator ions, to indicate that they are present in the solution during the reaction, but take no part in it.
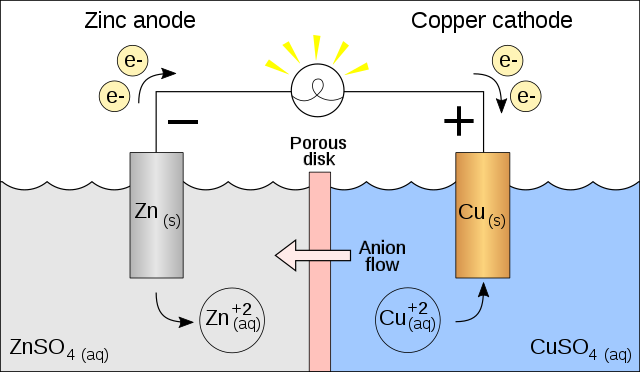
The overall redox reaction can be represented by two half-equations, one to show the reduction and the other to show the oxidation:
Oxidation half-equation: Mg(s) → Mg2+(aq) + 2e2-
Reduction half-equation: 2H+(aq) + 2e- → H2(g)
By combining these two half-equations, an ionic equation can be written, which does not show the spectator ions. For many redox reactions, it is possible to write two half-equations, one for the oxidation and the other for the reduction.
Working out the half-equations
Before writing down the two half-equations, the species being oxidized and the species being reduced must be established. This is best achieved using oxidation numbers. During oxidation, the oxidation number of an atom increases. During reduction, it decreases. The change in oxidation number also gives the number of electrons lost (oxidation) or gained (reduction).
Consider the reaction of aqueous iron (II) ions with acidified manganate(VI) ions. The overall ionic equation is:
5Fe2+(aq) + MnO4-(aq) + 8H+(aq) → 5Fe3+(aq) + Mn2+(aq) + 4H2O
The oxidation state of Fe changes from +2 to +3. This is oxidation and involves the loss of one electron. The oxidation state of Mn changes from +7 to +2. This is reduction and involves the gain of five electrons. We are now in a position to write the half-equations.
The oxidation involves conversion of Fe2+ and Fe3+ and is written as:
Fe2+(aq) → Fe3+(aq) + e-
The reduction involves conversion of the manganate (VII) ion into the manganese (II) ion. This involves the gain of five electrons per manganate(VI) ion:
5e- + MnO4-(aq) + 8H+(aq) → Mn2+(aq) + 4H2O(l)
Note that the half-equations are balanced, both in terms of their symbols and in terms of the charge. Note also that the aqueous hydrogen ions are not spectator ions, but are needed for the reduction to occur, and that the hydrogen ion is not itself reduced.
Then comes the transfer of electrons
The two half-equations clearly demonstrate the electron-transfer processes that redox reaction. The processes shown by the half-equations cannot take place independently, because reduction cannot occur without the simultaneous occurrence of oxidation. It is best to think of the half-equations as a ‘book-keeping’ exercise to show where electrons are lost and where they are gained.
Electrolysis reactions
Electrolysis can be defined thus: Electrolysis is the decomposition of a liquid by the passage of an electric current, which enters and leaves the compound through electrodes. Electrolysis is necessarily a redox reaction, since it involves electron transfer. The reactions represented by the two half-equations occur in different parts of an electrolytic cell. Reduction always occurs at the cathode (negative electrode) and oxidation always occurs at the anode (positive electrode). Consider the electrolysis of molten lead (II) iodide. The two half-equations are:
Oxidation half-equation: 2I- → I2 + 2e- occurs at the anode
Reduction half-equation: Pb2+ + 2e- → Pb occurs at the cathode
In electrolysis, these half-equations are referred to as electrode reactions.
So, what is an oxidizing agent?
An oxidising agent, or oxidant, is the substance or species that gains electrons during a redox reaction. It oxidises another substance by gaining electrons from it. So an oxidising agent is reduced during a redox reaction. Typically, oxidising agents are non-metal elements or compounds that have an element in a high, positive oxidation state. For example, fluorine is an oxidising agent, as is potassium dichromate (VI) in which chromium has an oxidation state of +6. In every reaction, the oxidising agent gains electrons. Fluorine molecules gain electrons to form fluoride ions, and dichromate (VI) ions gains electrons to form chromium (II) ions:
F2 + 2e- → 2F-
Cr2O72- + 14H+ + 6e- → 2Cr3+ + 7H2O
Note that these two half-equations are written to show that the oxidizing agent gains electrons. Sometimes, we want to compare the oxidising power of substances. That is, we want to compare the ability of oxidising agents to gain electrons. For example, fluorine is the better oxidising agent of the two shown above, but it is impossible to tell just from the half-equation.
What is a reducing agent?
A reducing agent, or reductant, is a substance or species that loses electrons during a redox reaction. It reduces another substance by losing electrons to it. So a reducing agent is oxidised during a redox reaction. Typically, reducing agents are metal elements or compounds that have an element in a low, positive oxidation state or a negative oxidation state. For example, potassium is a reducing agent, as is the sulfide ion in which sulfur has an oxidation state of -2. In every reaction, the reducing agent loses electrons. A potassium atom loses an electron to form a potassium ion, and a sulfide ion loses two electrons to form a sulfur atom.
K → K+ + e-
S2- → S + 2e-
Note that the two half-equations are written to show that the reducing agent loses electrons. Sometimes, we want to compare the reducing power of substances. That is. We want to compare the ability of reducing agents to lose electrons. For example, potassium is the better reducing agent of the two shown above, but it is impossible to tell this just from the half-equations.
SIMPLE CELLS
It has been known for more than two centuries that a potential difference is generated when two dissimilar metal electrodes are put into an electrolyte. What takes place in this so-called electrochemical cell is a redox reaction, in which oxidation occurs at one electrode and reduction at the other. This poses a question, since in a redox reaction an electron transfer must occur. Given that the two half-reactions (oxidation and reduction) are at different places, the transfer of electrons cannot take place during collision between the oxidising and the reducing agents. So where does it take place? Instead, the electrons are transferred via an external circuit (a metal wire). This may be regarded as electrolysis in reverse. Instead of a reaction occurring because of the passage of an electric current, an electric current (electron transfer) is produced because a redox reaction is occurring.
DANIELL CELL
In 1836. The English chemist John Fredrick Daniell constructed a simple battery that employed this idea. He used zinc and copper plates as the electrodes and two different electrolytes – aqueous copper (II) sulfate and aqueous zinc sulfate. (It is now known that in the Daniell cell zinc is in equilibrium with aqueous zinc ions and copper is in equilibrium with copper (II) ions.) When the two metal plates are connected by a metal wire, a current flows through the wire. The current is a result of the gain and loss of electrons that occur in the cell. During the operation of the cell, the zinc plate forms zinc ions and loses electrons. This corresponds to the oxidation half-equation. Meanwhile, at the copper plate, copper (I) ions gain electrons to form copper. This corresponds to the reduction half-equation.
Now, a redox reaction can occur only if electrons can be transferred. Only when the two plates are connected by a metal wire can this happen. The zinc plate, at which oxidation takes place, is the anode; the copper, at which reduction takes place is the cathode. The overall reaction that occurs in the cell is a combination of the two half-equations:
When zinc is placed directly into aqueous copper (II) sulfate, the same reaction takes place, but the electron transfer occurs during a collision between a zinc atom and a copper (II) ion.
ANOTHER SIMPLE CELL
It is possible to arrange the reaction between magnesium and hydrochloric acid as two half-reactions that occur in separate places, with the electrons being transferred via an external metal wire. The arrangement is shown in the figure below. Note the salt bridge.
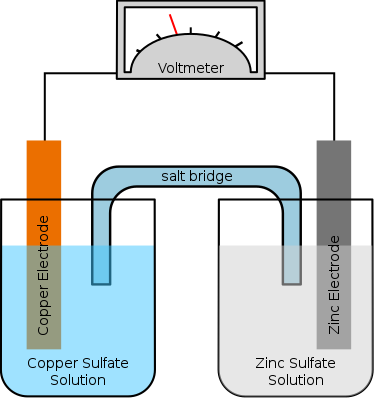
This allows the ions in the two electrolytes to migrate from one half-cell to the other without the electrolytes themselves mixing. Without a salt bridge, there would not be a complete circuit for the charge to flow round, and so no current would be produced. A salt bridge can be as simple as a piece of filter paper dipped in saturated aqueous potassium nitrate. Potassium nitrate is usually used because it does not react with most of the electrolytes used in electrochemical cells.
The understanding of cell potential and electrode potential is also crucial in the chemistry of an electric car. All these and many more would be discussed in my next post.
Thank you for coming.
REFERENCES
- https://en.wikipedia.org/wiki/Electric_car
- https://en.wikipedia.org/wiki/Redox
- https://www.khanacademy.org/science/chemistry/oxidation-reduction/redox-oxidation-reduction/a/oxidation-reduction-redox-reactions
- https://byjus.com/jee/redox-reactions/#:~:text=Redox%20reactions%20are%20oxidation%2Dreduction,process%20and%20an%20oxidation%20process.
- https://chem.libretexts.org/Bookshelves/Analytical_Chemistry/Supplemental_Modules_
- https://en.wikipedia.org/wiki/Electrolysis#:~:text=In%20chemistry%20and%20manufacturing%2C%20electrolysis,ores%20using%20an%20electrolytic%20cell.
- https://en.wikipedia.org/wiki/Oxidizing_agent
- https://www.thoughtco.com/definition-of-oxidizing-agent-605459#:~:text=An%20oxidizing%20agent%20is%20a,is%20thus%20an%20electron%20acceptor.
- https://byjus.com/chemistry/reducing-agent/
- https://chem.libretexts.org/Bookshelves/Analytical_Chemistry/Supplemental_Modules_
- https://en.wikipedia.org/wiki/Reducing_agent
- https://www.sciencedirect.com/topics/medicine-and-dentistry/primary-cell
- https://en.wikipedia.org/wiki/Primary_cell
- https://byjus.com/chemistry/daniell-cell/
- https://en.wikipedia.org/wiki/Daniell_cell
Thanks for your contribution to the STEMsocial community. Feel free to join us on discord to get to know the rest of us!
Please consider supporting our funding proposal, approving our witness (@stem.witness) or delegating to the @stemsocial account (for some ROI).
Thanks for using the STEMsocial app, which gives you stronger support. Including @stemsocial as a beneficiary could yield even more support next time.
Congratulations @empressteemah! You have completed the following achievement on the Hive blockchain and have been rewarded with new badge(s) :
You can view your badges on your board And compare to others on the Ranking
If you no longer want to receive notifications, reply to this comment with the word
STOP
Do not miss the last post from @hivebuzz:
Congratulations @empressteemah! You received a personal badge!
You can view your badges on your board and compare yourself to others in the Ranking
Do not miss the last post from @hivebuzz: